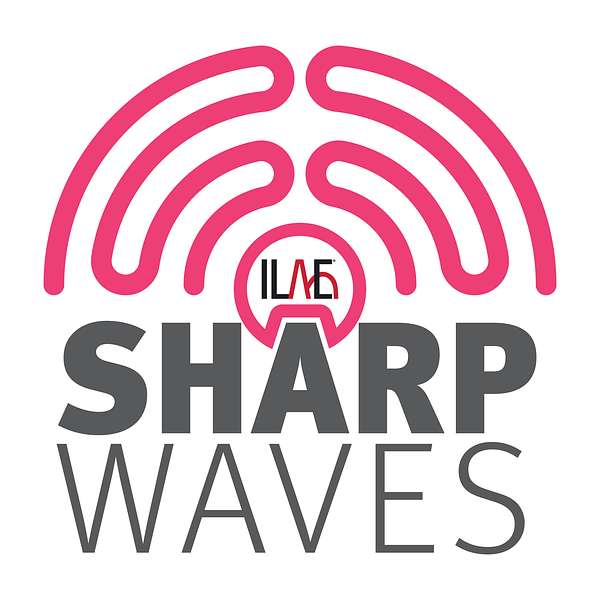
Sharp Waves: ILAE's epilepsy podcast
Sharp Waves: ILAE's epilepsy podcast
Astrocytes and epilepsy: Dr. Peter Bedner
Previously thought to be mere connective tissue and support for neurons, astrocytes are now understood to have many functions in the nervous system that are linked with seizures and epilepsy. Dr. Cecilie Nome spoke with Dr. Peter Bedner about astrocytes' involvement in epilepsy and how identifying and resolving astrocyte dysfunction is a new frontier in drug development.
Relevant articles:
de Ceglia R., et al. 2023. Specialized astrocytes mediate glutamatergic gliotransmission in the CNS. Nature 622, 120–129.
Grote A., et al. 2023. ‘Hippocampal innate inflammatory gliosis only’ in pharmacoresistant temporal lobe epilepsy. Brain 146(2), 549-560.
Ravi V.M., et al. 2015. Astrocyte uncoupling as a cause of human temporal lobe epilepsy. 2015. Brain 138(5), 1208-1222.
Sharp Waves episodes are meant for informational purposes only, and not as clinical or medical advice.
Let us know how we're doing: podcast@ilae.org.
The International League Against Epilepsy is the world's preeminent association of health professionals and scientists, working toward a world where no person's life is limited by epilepsy. Visit us on Facebook, X (Twitter), Instagram, and LinkedIn.
[00:00:00] Dr. Cecilie Nome: Welcome everyone to this podcast episode. Today we will be talking about astrocytes and epilepsy. My name is Cecilie Nome, and today we have Peter Bender with us here. Peter, could you please introduce yourself?
[00:00:17] Dr. Peter Bedner: I'm a senior postdoc at the Institute of Cellular and Neurosciences in Bonn in Germany, University of Bonn. And I'm working on epilepsy with a special focus on astrocyte dysfunction in epilepsy since 2006, almost 18 years. I'm happy to be here today.
[00:00:41] Dr. Cecilie Nome: We are very happy to have you here today. You've been working many years with astrocytes and have a lot of experience, so we're looking into looking forward to some interesting discussions. But to start, what is an astrocyte and what role do these cells play in the brain?
[00:01:01] Dr. Peter Bedner: Yeah, so astrocytes are a class of glial cells, which are non-neuronal cells in the central nervous system that do not produce electric impulses. They make up about half of the total volume of the human brain. Astrocytes are named after the Greek word astro, which means star, because they are star shaped, with long branching processes that make contact with blood vessels and neurons.
Initially, actually, glial cells, including astrocytes, were thought to merely form a connective tissue providing structural support to neurons. But nowadays, we know that astrocytes have a plethora of important functions which are crucial for proper functioning of the brain. So their function includes, for example, the supply of nutrients to neurons; they take up glucose from the bloodstream, metabolize it into lactate, and transport it to the neurons as an energy substrate.
They maintain the ion homeostasis. Astrocytes regulate the concentration of extracellular potassium, which is vital for maintaining the proper electrical activity of neurons and preventing hyperexcitability that can lead to seizures. They also regulate the neurotransmitters, as I said, take up excess neurotransmitters from the synaptic cleft and thereby prevent neuronal overexcitation which can lead to neuronal damage or death.
They also recycle the neurotransmitters to ensure efficient synaptic transmission and maintaining the balance of synaptic signaling. Astrocytes also help to form and maintain the blood-brain barrier and regulate the cerebral blood flow by signaling to blood vessels, to ensure adequate supplies of oxygen and nutrients in response to neuronal activity.
They also contribute to the immune response by release of cytokines and chemokines. And importantly, astrocytes also directly modulate synaptic transmission and plasticity through the release of so-called glial transmitters, such as ATP, glutamate, and D-serine.
Astrocytes are highly interconnected. They are connected to each other by large channels, the so-called gap junctions, which allow cell-to-cell diffusion of ions and small molecules like amino acids, sugar molecules, and nucleotides. So due to this gap junctional coupling, astrocytes form large syncytium-like functional networks that overlap with neuronal synaptic networks and enable their coordinated regulation and synchronization.
[00:03:45] Dr. Cecilie Nome: Yeah, that's a lot of different functions that these cells are having. And as you say, they also work together. So they're connected, if I understand you correctly, and then work together in the brain.
[00:04:00] Dr. Peter Bedner: Yeah.
[00:04:01] Dr. Cecilie Nome: Are there different types of astrocytes, and if so, what differentiates them?
[00:04:07] Dr. Peter Bedner: Astrocytes are a highly heterogeneous class of cells unified by their common neuroepithelial origin and their common functions. So they differ not only across brain regions, but also within the same brain region, in their morphology, gene expression profile, physiological properties, function, and response to injury and disease. For example, in a recent publication by Andrea Volterra and colleagues from Switzerland, they have shown that in the hippocampus, only a subpopulation of astrocytes possess machinery for calcium-dependent release of glutamate. So in the hippocampus there are astrocytes which are able to release glutamate and others which are not.
Based on their morphology and anatomical locations, astrocytes were classified in two main groups, protoplasmic and fibrous astrocytes. The protoplasmic astrocytes are primarily found in the gray matter of the brain and spinal cord and are characterized by many short, branched processes, and fibrous astrocytes are predominantly located in the white matter along myelinated nerve fibers of the brain and spinal cord and have long unbranched processes.
[00:05:21] Dr. Cecilie Nome: Thank you. So that's amazing that there are so many different subtypes. I think just some years ago, I learned that it was one or maybe two, two types of astrocytes, but it seems there's been a lot going on. These cells, they're doing so many different things. As you say, they're coupled together and they work as a syncytium and there are many different subtypes. But how are astrocytes involved in, in seizures and in epilepsy?
[00:05:50] Dr. Peter Bedner: So, of course, due to the central role of the astrocyte networks, neuronal functions and brain homeostasis, it's not surprising that various neurological disorders, including epilepsy, have been linked to alterations in astrocyte function.
There is growing evidence that many key regulatory functions of astrocytes are dysregulated in the epileptic brain and may contribute to the development and progression of the disease.
For example, impaired glutamate uptake has been shown in different models. As mentioned before, astrocytes play a vital role in regulating the levels of excitatory neurotransmitter glutamate in the synaptic cleft to prevent accumulation of glutamate in this extracellular space. Glutamate is taken up by astrocytes via specialized transporters and converted to glutamine by the enzyme glutamine synthetase and then shuttled back to neurons for glutamate free synthesis. So in epilepsy, reduced expression or dysfunction of both the glutamate transporter as well as the glutamine synthetase has been found, which of course leads to epilepsy. Excess glutamate in the external space can cause neuronal damage and promote seizures.
In addition, the abnormal release of excitatory neurotransmitters, such as ATP and glutamate, has been shown in different models, which enhances synaptic transmission and also contributes to hyperexcitability.
Another epilepsy-relevant astrocyte dysfunction which has been found in human and in experimental temporal lobe epilepsy is a loss of gap junctional coupling between astrocytes, and therefore the disruption of this astrocyte network. An important epilepsy-promoting functional consequence of this disruption is a reduced capacity to buffer elevated extracellular potassium concentrations during high neuronal activity.
In this process, which is known as spatial potassium buffering, the excess neuronally released extracellular potassium is passively taken out by astrocytes and distributed in the gap junction coupled network and released at sites of lower extracellular concentration. This process cannot be sustained in the absence of coupling, resulting in elevated extracellular potassium concentration and therefore in enhanced neuron excitability.
Another example is overexpression of astrocytic enzyme called adenosine kinase, which is actually also a hallmark of human experimental temporal lobe epilepsy. So this enzyme phosphorylates adenosine into adenosine monophosphate, a process that regulates intracellular and extracellular levels of adenosine. And adenosine in turn exerts powerful inhibitory effects on excitatory synaptic transmission, primarily through its interaction with presynaptic A1 adenosine receptors to suppress neurotransmitter release.
So overexpression of adenosine kinase would result in decreased adenosine concentration in the extracellular space and consequently enhance the excitability. This function may also call for breakdown of the blood brain barrier, which is often seen in the epileptic brain, especially in temporal lobe epilepsy. Due to this breakdown, immune cells and proteins from the blood can enter the brain and induce functional changes in astrocytes and neurons.
For example, blood serum albumin has been shown to be taken out by astrocytes, leading to transcriptional activation of inflammatory genes, downregulation of potassium channels, glutamate transporter, and gap junction proteins. So these astrocyte changes in turn promote the development of epilepsy. Astrocytes have also a key role in sustaining the neuroinflammatory response in epilepsy; they can secrete pro-inflammatory cytokines such as interleukin 1 beta or TNF alpha, and other inflammatory mediators, which can modify neuronal excitability and also promote seizures.
In addition, increased cytokine levels can trigger further astrocyte dysfunctions that lead to reduce glutamate uptake, reduce spatial buffering, and increased release of glutamate.
[00:10:17] Dr. Cecilie Nome: Thank you very much. When astrocytes change to all these different things that that affects them, they're usually called reactive astrocytes. So I'm wondering if you could tell me a little bit about reactive astrocytes and also astrogliosis, both what it is and how it's linked to epilepsy.
[00:10:40] Dr. Peter Bedner: Yeah, so astrogliosis, also called reactive astrocytes or reactive astrocytosis, are astrocytes that undergo morphological molecular and functional changes in response to pathological situations in the surrounding tissue. For example, CNS disease, injury, or brain infections.
So the key features of astrogliosis, first of all, they change their morphology. So they are getting hypertrophic. The astrocytes increase in size and their processes become more prominent, they proliferate. There is increased number of astrocytes.
Then they show molecular and functional changes. One example is upregulation of the glial fibrillary acidic protein, which is commonly used as a marker of astrocyte activation. They also show altered gene expression, so they express different sets of genes, including those involved in inflammation, neurotransmitter regulation, and cellular repair mechanisms.
They release signaling molecules such as cytokines or chemokines, which can modulate inflammation and interact with other cell types in the brain. And of course, in case of severe chronic injury, astrocytes can form a dense fibrous network known as glial scar. This structure can help to isolate the injury inside, but also may also impede regeneration and proper neuronal functions.
So astrogliosis initially serves as protective, it has a protective role by attempting to maintain a central nervous system homeostasis, but persistent astrogliosis can contribute to the development and progression of seizures and epilepsy by altering neuronal excitability, disrupting neurotransmitter balance, and promoting neuroinflammation.
[00:12:32] Dr. Cecilie Nome: So like you say, astrogliosis could be a protective thing, but at some point it can also lead to epilepsy. So it's like two different aspects of astrogliosis. I guess this has been studied in several different types of epilepsy, but are there any specific types of epilepsy where astrogliosis is more commonly observed?
[00:12:54] Dr. Peter Bedner: Actually, astrogliosis is commonly observed in several types of focal epilepsy, particularly those associated with structural abnormalities chronic injury or significant inflammation. So it's a pathological hallmark, for example, of temporal lobe epilepsy with hippocampal sclerosis, of focal cortical dysplasia, of post traumatic epilepsy, stroke-related epilepsy and Rasmussen's encephalitis. But, for example, there is no significant gliosis in in genetic focal epilepsies.
[00:13:25] Dr. Cecilie Nome: So like you say, gliosis can then develop in relation to many different disorders, like stroke, you mentioned, and also brain infections. And I think also there have been some studies in dementia, actually. But, but not all these patients develop epilepsy. Why not?
[00:13:43] Dr. Peter Bedner: So the development of epilepsy in context of gliosis is a multifactorial process influenced by the severity and type of brain injury, the specific neuronal networks involved, individual patient characteristics, and the nature of the underlying condition. Important is that there is no prototypical reactive astrocytes, so reactive astrocytes may adopt multiple states depending on the context, with only a fraction of common changes between different states.
Some forms of reactive astrogliosis are associated with neuroprotective effects and maintenance of homeostasis, while others are linked to pro-epileptic changes, such as altered neurotransmitter regulation and disruption of ion balance.
In addition, the time course of gliosis is important. Acute reactive gliosis might initially serve a protective role by stabilizing the environment after injury. Chronic causes can lead to persistent changes in brain tissue which can favor seizures. So the duration and persistence of gliosis can therefore determine whether it leads to epilepsy.
Finally, the severity and precise location of the brain injury or disease play a crucial role in determining whether gliosis will contribute to epileptogenesis.
[00:15:06] Dr. Cecilie Nome: This leads to my next question, because last year there was a paper published by Alexander [Grote] et al in Brain and they found that patients with temporal lobe epilepsy and hippocampal sclerosis, they seem to exhibit different types of gliosis and have reactive astrocytes that seem to have different features in these two different types. What are your thoughts on this finding?
[00:15:33] Dr. Peter Bedner: Yeah, so this is indeed a very interesting paper showing that reactive astrocytes can exist in multiple states rather than fitting into a single prototypic form. Alexander Grote and colleagues showed that reactive astrocytes in the so called “gliosis only subtype” of temporal lobe epilepsy, in which the primary pathological finding is reactive gliosis without significant neuronal loss or other structural abnormalities, show an upregulation of inflammatory proteins. Whereas reactive astrocytes in hippocampal sclerosis, which shows neuronal loss and other histopathological changes have astrocytes with non-inflammatory states. Actually, we also showed in 2015 that in hippocampal sclerosis, the astrocytes are not anymore coupled by gap junctions; the astroglial syncytium is completely disrupted in the hippocampal sclerosis, but not in non-sclerotic tissue.
So we think that we've found in the animal models that this uncoupling occurs very early in epileptogenesis and might be actually the primary cause of temporal lobe epilepsy with hippocampal sclerosis.
But of course, in a non-sclerotic hippocampus, the coupling is intact. So this shows that the astrocytes or extra reactive astrocytes can have different properties. And therefore also the mechanisms by which they may promote epilepsy are likely to be different.
[00:17:06] Dr. Cecilie Nome: That's very interesting. And I think it's also very interesting like you said, that the study you published in 2015 also shows some of the same things and that there are maybe different types of gliosis and there are many different factors that probably can cause different types, and that this also was found in the recent paper by Alexander Grote. Very interesting.
Yeah, so now we have discussed how gliosis can cause seizures. But I have also read that seizures can worsen and or even induce gliosis. So how are these two processes connected?
[00:17:45] Dr. Peter Bedner: Yes, of course. Seizures cause neuronal damage and metabolic stress, and damaged neurons or stressed neurons actually can release so called damage-associated molecules.
So there is a molecule, for example, called high-mobility group box 1 protein, which can bind to astrocytes and which can activate the astrocytes. Also, activated microglial seizures activate microglia, which then release pro-inflammatory cytokines, which then can further activate astrocytes and promote astrogliosis.
Furthermore, the high metabolic activity during seizures generates reactive oxygen species and other free radicals which cause oxidative stress, which also triggers astrogliosis. And also seizures can cause high excess glutamate levels and intracellular potassium accumulation, which is also a trigger for astrogliosis. And yeah, finally, seizures can induce blood-brain barrier disruption and blood-derived proteins enter the brain and may also induce astrogliosis. So then the astrogliosis in turn further promotes or intensifies epileptogenesis in that case.
[00:18:55] Dr. Cecilie Nome: Thanks.
Are there any medications available today that can reverse or even halt the progressions of gliosis in humans? Or are there any promising drug candidates being studied?
[00:19:10] Dr. Peter Bedner: Unfortunately, there is no current medication that can fully reverse gliosis in humans, but some drugs and experimental therapies show promise in reducing or halting its progression.
So first of all the commonly used anti-epileptic drug levetiracetam has shown anti-inflammatory effects in some models, suggesting it might reduce gliosis. It's often used in epilepsy, but its specific impact on gliosis in humans remains unclear so far. Also, some anti-inflammatory drugs show potential in reducing gliosis in preclinical models, but their efficiency and in treating chronic gliosis and epilepsy in humans is still under investigation.
Then promising drug candidates are, for example, minocycline, which is an antibiotic with anti-inflammatory properties. Minocycline has been shown to reduce microglia activation and astrogliosis in animal models of epilepsy. Also cannabinoids, which have been shown to reduce astrocytes reactivity and gliosis in epilepsy models.
And another example is the mTOR inhibitor rapamycin, that has been shown to reduce gliosis and seizures in animal models of epilepsy.
But it's important to note that it's probably not a useful therapeutic strategy to completely inhibit gliosis levels, as this process involves not only harmful but also beneficial astrocyte changes. So, useful therapeutic strategies must therefore aim to maintain or enhance the beneficial effects of astrogliosis, while blocking or reducing the harmful ones. Those components that specifically target for epileptic astrocyte dysfunction, such as impaired astrocytic glutamate uptake or impaired gap junctional coupling between astrocytes, may be promising candidates for actually more efficient and specific anti-epileptic drugs.
[00:21:13] Dr. Cecilie Nome: Do you think we will be able to inhibit or stop the development of gliosis in the future, or to inhibit or stop one of those features that you talked about that are negative for the brain?
[00:21:27] Dr. Peter Bedner: Actually, the role of astrocyte dysfunction and its potential as a target for new anti-epileptic drugs is an area of intense research at present. But I think a deeper understanding of the mechanism and the role of astrogliosis in epileptogenesis is needed to develop new astrocyte directed therapeutic strategies for the treatment of seizures and epilepsy.
[00:21:50] Dr. Cecilie Nome: Thank you so much for participating in our podcast episode today. It's been a pleasure to have you here and, and to learn all the things about astrocytes, since you are so experienced in this topic. And so thank you very much.
[00:22:05] Dr. Peter Bedner: Thank you very much.